The role of neuroinflammation in the progression of Parkinson’s Disease
Author: Alex Tomceac
Download: [ PDF ]
Neuroscience In Review
Parkinson’s disease (PD) is a progressive neurodegenerative disease characterized by the loss of dopaminergic neurons (DA) in the substantia nigra of the brain. PD is primarily characterized by Lewy body formations- clumps of misfolded proteins found inside neurons. The Lewy bodies are composed as a result of the accumulation of aggregated alpha-synuclein (a-syn) and these aggregates interfere with the normal functions of the cell. Overwhelming evidence in research has shown that a-syn is responsible for the progression of Parkinson’s disease. In recent years, studies have shown a-syn does not only affect neuronal processes which disrupt the homeostatic environment of dopaminergic neurons, but also directly activates the immune system by producing an excessive inflammatory response in the brain through microglia and astrocyte activation. One study has shown abnormal levels of two pro-inflammatory cytokines, interleukin (IL-1), and tumor necrosis factor-a (TNF) in PD patients implying the major role that the immune system might play on the progression of the disease. This literature review will focus on neuroinflammation-- specifically on microglia and astrocyte activation in relation to the aggregation of a-syn in PD pathology, and the potential of drugs that can target astrocytes and microglia in order to reduce the hyperreactivity of these cells in response to a-syn aggregation.
Introduction
Parkinson’s disease (PD) is one of the most common neurodegenerative disorders in the world. It is estimated that more than 10 million people, globally, are affected by PD (Parkinson’s Foundation, 2021). PD is characterized by the loss of dopaminergic neurons in the nigrostriatal pathway which involves the substantia nigra pars compacta (SNpc) and other dopaminergic pathways outside of this circuit. The slow degeneration of dopaminergic neurons causes dysfunction in motor symptoms including bradykinesia, tremors, and rigidity (Javed et al.,2020). Furthermore, PD is also characterized by misfolded protein inclusions, known as Lewy bodies, which are abundantly present in neurons (Murphy et al 2011). Despite extensive research over the years in PD pathogenesis, the role of misfolded proteins is not fully understood, however; multiple pathogenic mechanisms have been proposed to explain neuronal degeneration including mitochondrial dysfunction, oxidative stress, abnormal protein degradation, and excitotoxicity (Kim et al., 2007). Neuroinflammation has also been proposed to play a role in pathogenesis and progression of PD (Stephenson et al., 2018). Some mechanisms have been proposed including microglial-mediated activation and astrogliosis (Figure 1). Under normal conditions, microglial cells and astrocytes provide a neuroprotective role to neurons. However, in PD pathogenesis these supportive cells contribute to the progression of the disease. Studies have shown that activation of microglia is beneficial in the early stages of PD, but as the disease progresses, the overactivation and continuous cycle of neuroinflammatory response becomes cytotoxic to the the brain, causing dopaminergic neurons to die (Zeng et al., 2005). It must be noted that inflammatory responses are not specific to PD. Other neurodegenerative diseases, including amyotrophic lateral sclerosis, Alzheimer’s, and Huntington’s disease are also characterized by neuroinflammation (Stephenson et al., 2018). This evidence suggests that activation of microglial is not specific to PD but rather it is a contributing factor to progression of the disease with other supporting causes. In this review, I highlight the link between neuroinflammation and PD progression with emphasis on microglial activation, astrogliosis, and potential neuroinflammatory targets which promote neuroprotective pathways in astrocytes.
Neuroinflammation by microglial activation in PD
Microglia are important support cells for neurons in the central nervous system and play a major role in mediating immune responses in the brain. The earliest post-mortem studies of PD patients showed reactive microglia as recognized elements in the PD pathology (McGreer et al., 1998). One potential hypothesis on why the SN is predominantly affected in PD is because there is a high concentration of resting microglia in this region (Lawson et al., 1990). Microglial activation has been identified in rodent models by injection of MPTP- a neurotoxin which induces Parkinsonism symptoms in monkeys and mice. After MPTP injections, microglial cells are activated and after 48 hours of exposure to the neurotoxin, death of dopaminergic neurons is observed. Moreover, injections with lipopolysaccharides (a bacteriotoxin which activates microglia) in rats leads to the release of increased levels of proinflammatory cytokines interleukin- 1β and TNF-α and reactive oxygen species (ROS) (Nishimura et al., 2001). The high concentrations of ROS and proinflammatory cytokines are cytotoxic to dopaminergic neurons which leads to cell death. In the early stages of PD, studies indicate microglia have protective mechanisms for neurons however, overactivation leads to cytotoxicity to neurons as PD progresses (Zeng et al., 2005). There are two microglial phenotypes expressed in the brain which are known as the M1 and M2 phenotypes. M1 activation is associated with increases of proinflammatory cytokines, while M2 activation leads to anti-inflammatory processes and promotes healing and tissue repair. In the initial stages of PD, the M2 phenotype is predominant and with age progression there is downregulation of epigenetic factors such as Jmjd3 which are essential for M2 microglia polarization. With the suppression of Jmjd3, the M2 microglia is inhibited and the M1 phenotype is over exaggerated, promoting neuronal cell death through the activation of proinflammatory cytokines (Tang et al., 2014). These findings suggest microglia-derived inflammation is a contributing factor to PD pathology and changes in microglia subtype implies that the cell function changes which occur with aging and progression. Moreover, microglia may induce astrocytes to lose its neurotrophic functions and promote a neurotoxic environment.
Neuroinflammation by astrogliosis
Astrocytes are the most abundant glial cell in the CNS and also support neurons by producing antioxidants and releasing neurotrophic factors; however, astrocytes produce proinflammatory factors which are detrimental in the long term to the survival of DA neurons in PD. It is known that neurons accumulate pathological a-syn protein, however astrocytes also accumulate and deposit a-syn which disrupts their normal functions, making them reactive (Saijo et al., 2009). Astrocytes also respond to inflammatory stimulations in rodent models by producing proinflammatory cytokines in the same fashion as microglia (Saijo et al., 2009). Glial fibrillary acidic protein (GFAP) is up regulated in reactive astrocytes and accumulation of GFAP-expressing astrocytes are increased in PD models compared to controls (Yamada et al.,1992). In the initial stages of PD, microglia are activated, but after a short period of time, these microglia cause A1 astrocyte activation via secretion of cytokines such as IL-1α and TNF. Upregulation of proinflammatory factors amplifies those signals to release more immune factors, leading to uncontrolled neuroinflammation due to the synergistic effect of microglia and astrocytes promoting cytokines and other factors.. Moreover, A1 type astrocytes induce neuronal death by upregulation of neurotoxic factors compared to A2 which are protective (Liddelow et al., 2017). In PD, the activation of type A1 astrocytes fails to protect neurons leading to neuronal death and neurodegeneration. Astrocytes also play an important role in the regulation of the blood-brain barrier (BBB) by assisting in the formation of tight junctions and regulating which molecules enter the brain. Astrocyte dysfunction causes disruptions of the BBB and increases the permeability of proinflammatory cytokines at the tight junctions. Gray et al. found PD patients to have increased the BBB permeability in the putamen which receives dopaminergic projections from the SN (Gray et al., 2015). Together these findings suggest astrocyte dysfunction leads to neurodegeneration of dopaminergic neurons and reactive astrogliosis in PD.
Future directions: Therapeutic targets for neuroinflammation
Most recent studies have focused on reducing neuroinflammation by upregulating neuroprotective pathways in astrocytes providing neuroprotection to dopaminergic neurons. Glutathione (GSH) is the most abundant antioxidant molecule found in the CNS which fights against reactive oxidative species (ROS) which are present in higher concentrations in PD patients. The Nrf2 signaling cascade in astrocytes was found to promote antioxidants such as GSH (Miyazaki et al., 2016). A 2009 study found that overexpression of Nrf2 reduces the neurotoxicity of MPTP in mouse models (Chen et al., 2009). Moreover, activation of Nrf2 pathways delayed alpha-synuclein aggregation and motor symptoms were alleviated in transgenic mice expressing human mutant a-syn strain (Gan et al., 2012). Antioxidative properties in astrocytes were upregulated by the Nrf2 pathway when the drug ritogotine (a 5-HT1A receptor agonist) was administered to mice (Isooka et al., 2020). In a different study, Yun et al., reported NLY01, a GLP1 receptor agonist, inhibits the conversion of astrocytes to the A1 neurotoxic phenotype in an α-syn PFF mouse model. Furthemore, NLY01 had protective properties against degeneration of dopaminergic neurons and behavioral deficits in the PD model (Yun et al., 2018). In conclusion, neuroprotective pathways in astrocytes can be activated by targeting the 5-HT1A receptor, upregulation of the Nrf2 pathway, and inhibition of A1 astrocyte phenotype.
Current therapeutic approaches have been focused on targeting the motor symptoms of PD rather than preventative therapies. Moreover, animal models are limited in reproducing the pathological features of PD in humans. In some mouse models, Lewy bodies are absent, suggesting these studies do not fully replicate the complex pathological system expressed in humans (Bezard et al., 2011). Animal models are limited in their results because they focus on specific components of PD which may not effectively translate to the diverse human pathology. New approaches to study PD in humans can utilize human-derived stem cell cultures and induced pluripotent stem cells to translate into effective treatments to those who suffer from PD (Marshall et al., 2019).
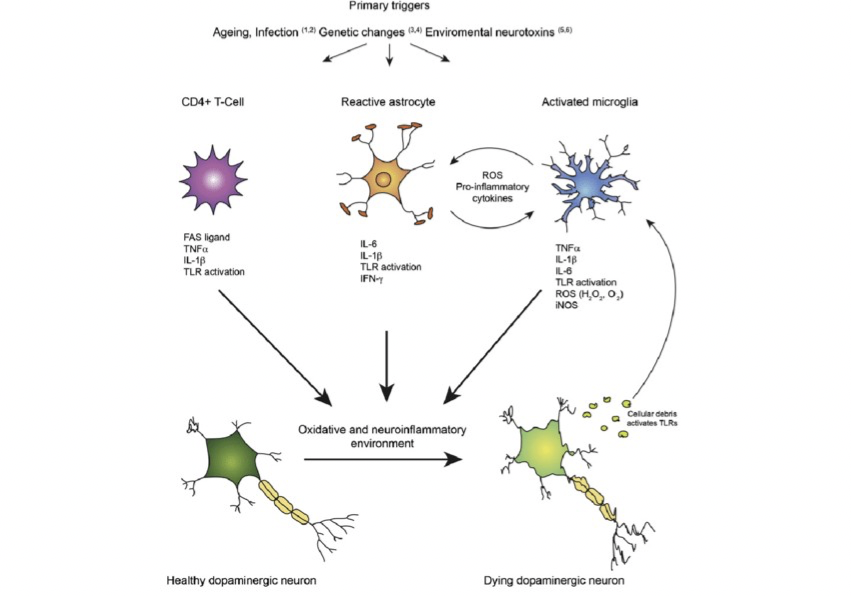
[+] References
Saijo, K., Winner, B., Carson, C. T., Collier, J. G., Boyer, L., Rosenfeld, M. G., ... & Glass, C. K. (2009). A Nurr1/CoREST pathway in microglia and astrocytes protects dopaminergic neurons from inflammation-induced death. Cell, 137(1), 47-59.
Gray, M. T., & Woulfe, J. M. (2015). Striatal blood–brain barrier permeability in Parkinson's disease. Journal of Cerebral Blood Flow & Metabolism, 35(5), 747-750.
Liddelow, S. A., Guttenplan, K. A., Clarke, L. E., Bennett, F. C., Bohlen, C. J., Schirmer, L., ... & Barres, B. A. (2017). Neurotoxic reactive astrocytes are induced by activated microglia. Nature, 541(7638), 481-487.
Gan, L., Vargas, M. R., Johnson, D. A., & Johnson, J. A. (2012). Astrocyte-specific overexpression of Nrf2 delays motor pathology and synuclein aggregation throughout the CNS in the alpha-synuclein mutant (A53T) mouse model. Journal of Neuroscience, 32(49), 17775-17787.
Isooka, N., Miyazaki, I., Kikuoka, R., Wada, K., Nakayama, E., Shin, K., ... & Asanuma, M. (2020). Dopaminergic neuroprotective effects of rotigotine via 5-HT1A receptors: possibly involvement of metallothionein expression in astrocytes. Neurochemistry international, 132, 104608.
Yun, S. P., Kam, T. I., Panicker, N., Kim, S., Oh, Y., Park, J. S., ... & Ko, H. S. (2019) Block of A1 astrocyte conversion by microglia is neuroprotective in models of Parkinson's disease. Nature medicine, 24(7), 931-938.
Miyazaki, I., Murakami, S., Torigoe, N., Kitamura, Y., & Asanuma, M. (2016). Neuroprotective effects of levetiracetam target xCT in astrocytes in parkinsonian mice. Journal of neurochemistry, 136(1), 194-204.
Yamada, T., McGeer, P. L., & McGeer, E. G. (1992). Appearance of paired nucleated, Tau-positive glia in patients with progressive supranuclear palsy brain tissue. Neuroscience letters, 135(1), 99-102.
Saijo, K., Winner, B., Carson, C. T., Collier, J. G., Boyer, L., Rosenfeld, M. G., ... & Glass, C. K. (2009). A Nurr1/CoREST pathway in microglia and astrocytes protects dopaminergic neurons from inflammation-induced death. Cell, 137(1), 47-59.
Lawson, L. J., Perry, V. H., Dri, P., & Gordon, S. (1990). Heterogeneity in the distribution and morphology of microglia in the normal adult mouse brain. Neuroscience, 39(1), 151-170.
McGeer, P. L., Itagaki, S., Boyes, B. E., & McGeer, E. G. (1988). Reactive microglia are positive for HLA‐DR in the substantia nigra of Parkinson's and Alzheimer's disease brains. Neurology, 38(8), 1285-1285.
Nishimura, M., Mizuta, I., Mizuta, E., Yamasaki, S., Ohta, M., Kaji, R., & Kuno, S. (2001). Tumor necrosis factor gene polymorphisms in patients with sporadic Parkinson's disease. Neuroscience letters, 311(1), 1-4.
Zeng, H. Y., Zhu, X. A., Zhang, C., Yang, L. P., Wu, L. M., & Tso, M. O. (2005). Identification of sequential events and factors associated with microglial activation, migration, and cytotoxicity in retinal degeneration in rd mice. Investigative ophthamology & visual science, 46(8), 2992-2999.
Tang, Y., Li, T., Li, J., Yang, J., Liu, H., Zhang, X. J., & Le, W. (2014). Jmjd3 is essential for the epigenetic modulation of microglia phenotypes in the immune pathogenesis of Parkinson’s disease. Cell Death & Differentiation, 21(3), 369-380.
Javed, H., Thangavel, R., Selvakumar, G. P., Dubova, I., Schwartz, N., Ahmed, M. E., ... & Khan, M. M. (2020). NLRP3 inflammasome and glia maturation factor coordinately regulate neuroinflammation and neuronal loss in MPTP mouse model of Parkinson’s disease. International immunopharmacology, 83, 106441.
Volpicelli-Daley, L. A., Luk, K. C., Patel, T. P., Tanik, S. A., Riddle, D. M., Stieber, A., ... & Lee, V. M. Y. (2011). Exogenous α-synuclein fibrils induce Lewy body pathology leading to synaptic dysfunction and neuron death. Neuron, 72(1), 57-71.
Taylor, J. M., Main, B. S., & Crack, P. J. (2013). Neuroinflammation and oxidative stress: co-conspirators in the pathology of Parkinson’s disease. Neurochemistry international, 62(5), 803-819.
Bezard, E., & Przedborski, S. (2011). A tale on animal models of Parkinson's disease. Movement Disorders, 26(6), 993-1002.
Marshall, L. J., & Willett, C. (2018). Parkinson’s disease research: Adopting a more human perspective to accelerate advances. Drug discovery today, 23(12), 1950-1961.
[+] Other Work By Alex Tomceac
Opening the blood-brain barrier
Neuroanatomy
The study examines a novel myelin imaging technique (REMyDI) that was used to discover the amount of myelin in patients with multiple sclerosis and how the quantity was correlated with their physical and cognitive disability ratings.
Plasticizers Disrupt Neuro Signaling in Adult Brains
Neurophysiology
Readily used plasticizers such as BPA and BPS alter neurotransmission in the brains of goldfish.